Introduction
Who does not know about COVID-19 pandemic? The COVID-19 pandemic, caused by the SARS-CoV-2 virus, has had a profound impact globally since it began in late 2019. As of now, the COVID-19 pandemic has resulted in over 6 million reported deaths worldwide. However, the World Health Organization (WHO) estimates that the actual death toll, including both direct and indirect impacts, could be around 14.9 million between January 2020 and December 2021. COVID-19 is caused by the SARS-CoV-2 virus, which belongs to the Coronaviridae family.
Coronaviruses are a large family of viruses that can infect animals and humans. They are named for the crown-like spikes on their surface, which resemble a solar corona when viewed under a microscope. This viral family belongs to the order Nidovirales, family Coronaviridae, and the subfamily Orthocoronavirinae. The viruses within this family are enveloped and possess a single-stranded, positive-sense RNA genome, making them one of the largest RNA viruses known. Coronaviruses can cause a wide range of diseases, from the common cold to more severe conditions such as Severe Acute Respiratory Syndrome (SARS), Middle East Respiratory Syndrome (MERS), and the novel coronavirus disease (COVID-19). While some coronaviruses only cause mild, cold-like symptoms, others have the potential to cause severe respiratory and systemic infections, particularly in vulnerable populations such as the elderly, immunocompromised individuals, and those with pre-existing health conditions.
Brief history of Coronaviruses
The history of coronaviruses in scientific literature dates back to the 1930s, when the first coronavirus infections were identified in domesticated animals. These early discoveries were primarily related to veterinary medicine, where coronaviruses were found to cause diseases in poultry, cattle, pigs, and other livestock. For example, in 1931, a disease now known as avian infectious bronchitis virus (IBV) was discovered in chickens, causing respiratory illness and significant economic losses in the poultry industry. In the 1960s, coronaviruses were first identified in humans. Researchers isolated two strains, HCoV-229E and HCoV-OC43, from patients suffering from the common cold. These early human coronaviruses were recognized as common causes of mild upper respiratory tract infections, often associated with winter colds. For several decades, these two strains, along with a few others, were the only coronaviruses known to affect humans, and their impact was considered relatively minor compared to other respiratory viruses like influenza.
The perception of coronaviruses dramatically changed with the emergence of SARS-CoV in 2002. This novel coronavirus caused an outbreak of Severe Acute Respiratory Syndrome (SARS), which began in Guangdong Province, China, and quickly spread to other parts of Asia, Europe, and North America. The SARS outbreak infected over 8,000 people and resulted in nearly 800 deaths. This was the first time a coronavirus was recognized as a significant cause of severe human disease, and it highlighted the potential of coronaviruses to cause global health crises.Following the SARS outbreak, scientists intensified their efforts to study coronaviruses, leading to the identification of other coronaviruses in animals and humans. In 2012, another novel coronavirus, MERS-CoV, emerged in the Middle East, causing Middle East Respiratory Syndrome (MERS). MERS was even deadlier than SARS, with a mortality rate of around 34%, but it did not spread as widely, with most cases confined to the Arabian Peninsula.
In December 2019, the world was introduced to yet another coronavirus, SARS-CoV-2, responsible for the COVID-19 pandemic. The emergence of COVID-19 is a pivotal moment in modern public health history. The earliest cases of what would later be known as COVID-19 were reported in December 2019 in the city of Wuhan, the capital of Hubei Province in central China. Wuhan, a major transportation hub with a population of over 11 million people, became the epicenter of a mysterious outbreak of pneumonia cases. The first cluster of patients was linked to the Huanan Seafood Wholesale Market, which sold live wild animals in addition to seafood. This market setting suggested a zoonotic origin, where the virus might have been transmitted from animals to humans, similar to previous outbreaks of coronaviruses like SARS-CoV and MERS-CoV. Initial reports to the World Health Organization (WHO) described a novel pneumonia with symptoms including fever, cough, and difficulty breathing, which did not respond to standard treatments for known respiratory illnesses.
On December 31, 2019, Chinese authorities notified the WHO of the outbreak, marking the start of international awareness of the virus. In the following days, researchers in China quickly identified the causative agent as a novel coronavirus, which was named SARS-CoV-2 due to its genetic similarities to the SARS-CoV virus that caused the 2002 SARS outbreak. The disease caused by this virus was officially named COVID-19, an abbreviation for “Coronavirus Disease 2019.”
The rapid spread of COVID-19 beyond Wuhan was a critical factor in its emergence as a global pandemic. Despite efforts by Chinese authorities to contain the virus, including the unprecedented lockdown of Wuhan on January 23, 2020, the virus had already begun spreading to other parts of China and internationally. Early cases outside China were reported in Thailand, Japan, and South Korea, involving travelers who had recently visited Wuhan. The virus’s ability to spread efficiently from person to person, primarily through respiratory droplets and close contact, became evident as cases multiplied. By the end of January 2020, COVID-19 cases were confirmed in numerous countries across Asia, Europe, North America, and beyond. The WHO declared the outbreak a Public Health Emergency of International Concern (PHEIC) on January 30, 2020, acknowledging the global threat posed by the virus. As February 2020 progressed, COVID-19 spread to more countries, with significant outbreaks emerging in Italy, Iran, and South Korea. These developments demonstrated that the virus was no longer confined to China or its immediate neighbors. On March 11, 2020, the WHO officially declared COVID-19 a pandemic, highlighting the severity and global reach of the outbreak. This was the first pandemic caused by a coronavirus, and it led to widespread alarm as countries around the world grappled with how to respond.
Structure of the SARS-CoV-2 (COVID-19) Virus
The structure of SARS-CoV-2, the virus responsible for COVID-19, is integral to understanding how it infects human cells and causes disease. As a member of the coronavirus family, SARS-CoV-2 shares several structural characteristics with other coronaviruses, including SARS-CoV and MERS-CoV, but also has unique features that contribute to its pathogenicity and transmission. SARS-CoV-2 is an enveloped virus, meaning it has a lipid bilayer that encases its genetic material. The virus is roughly spherical, with a diameter of about 60-140 nanometers. It has a characteristic “crown-like” appearance under an electron microscope, which is due to the presence of spike (S) proteins projecting from its surface. These spike proteins play a crucial role in the virus’s ability to infect human cells.
The core of the virus contains its genetic material, which is a single-stranded positive-sense RNA (+ssRNA) genome. The genome of SARS-CoV-2 is approximately 29.9 kilobases in length, making it one of the largest RNA genomes among known viruses. This RNA genome encodes for several structural, non-structural, and accessory proteins that are essential for the virus’s replication and assembly. The RNA genome is encapsulated by the nucleocapsid (N) protein, which binds to the RNA in a helical structure. The N protein plays a crucial role in protecting the viral RNA and ensuring its correct packaging into new virions during replication.
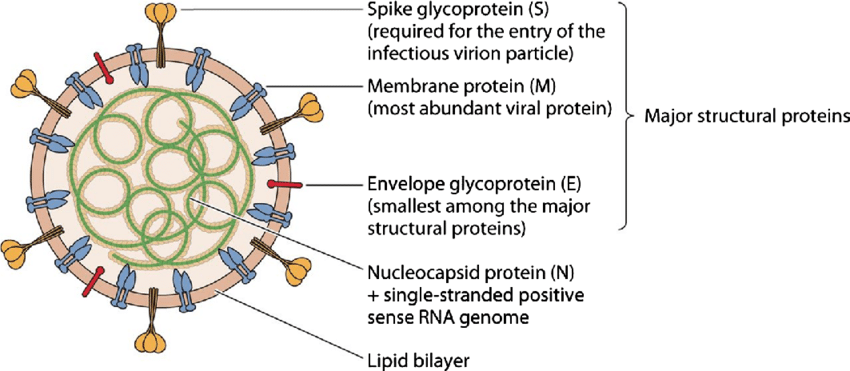
SARS-CoV-2 has four main structural proteins that are critical to its function and infectivity: the spike (S) protein, the envelope (E) protein, the membrane (M) protein, and the nucleocapsid (N) protein.
Spike (S) Protein
The spike protein is the most prominent feature of SARS-CoV-2 and is responsible for the virus’s ability to bind to and enter host cells. It is a transmembrane glycoprotein that forms homotrimers protruding from the viral surface. The S protein is divided into two functional subunits: S1 and S2.
- S1 Subunit: Contains the receptor-binding domain (RBD) that binds to the host cell receptor angiotensin-converting enzyme 2 (ACE2). The binding of the RBD to ACE2 is the first step in the viral entry process.
- S2 Subunit: Mediates the fusion of the viral membrane with the host cell membrane, allowing the viral RNA to enter the host cell cytoplasm. This fusion is triggered by proteolytic cleavage of the S protein at specific sites by host proteases such as furin and TMPRSS2.
Envelope (E) Protein
The E protein is a small, integral membrane protein involved in the assembly and release of new viral particles. It also plays a role in the virus’s ability to cause disease, possibly by modulating host cell processes and contributing to the pathogenicity of the virus.
Membrane (M) Protein
The M protein is the most abundant structural protein in the virus and is crucial for maintaining the shape of the viral envelope. It interacts with the other structural proteins to orchestrate the assembly of new virions and ensures the stability of the viral particle.
Nucleocapsid (N) Protein
As mentioned earlier, the N protein binds to the viral RNA genome and forms a protective nucleocapsid around it. This protein is essential for the replication and transcription of the viral RNA and is also involved in the assembly of new viral particles.
Lipid envelope
The lipid envelope of SARS-CoV-2 is derived from the host cell membrane during the viral assembly process. This envelope not only encases the viral components but also aids in the fusion of the virus with host cells, facilitating the release of the viral genome into the host cell. The lipid bilayer is embedded with the S, E, and M proteins, giving the virus its characteristic structure. The lipid envelope makes SARS-CoV-2 susceptible to inactivation by detergents, alcohol, and other lipid-solubilizing agents, which is why handwashing with soap and the use of alcohol-based hand sanitizers are effective measures in preventing the spread of the virus.
Accessory proteins
In addition to the four main structural proteins, the SARS-CoV-2 genome encodes several accessory proteins that are not directly involved in forming the viral particle but play roles in modulating the host immune response and enhancing viral replication. These include proteins such as ORF3a, ORF6, ORF7a, ORF7b, ORF8, and ORF10, among others. The precise functions of some of these accessory proteins are still being studied, but they are believed to help the virus evade the host immune system and adapt to the host environment.
Mutations and variants
SARS-CoV-2, like other RNA viruses, has a high mutation rate, which has led to the emergence of numerous variants. Some mutations occur in the spike protein, particularly in the receptor-binding domain (RBD), which can affect the virus’s transmissibility, virulence, and resistance to neutralizing antibodies. Variants such as Alpha, Beta, Gamma, Delta, and Omicron have shown significant mutations that have impacted global public health responses, influencing vaccine effectiveness, transmission rates, and treatment strategies.
Transmission and contagiousness of COVID-19
The transmission and contagiousness of COVID-19, caused by the SARS-CoV-2 virus, have been key factors in its rapid global spread and the challenges faced in controlling the pandemic. SARS-CoV-2 primarily spreads through several key mechanisms:
Respiratory droplets
The primary mode of transmission is through respiratory droplets. When an infected person coughs, sneezes, talks, or breathes heavily, they release droplets containing the virus into the air. These droplets can be inhaled by people nearby, typically within a range of about 1 to 2 meters (3 to 6 feet), leading to new infections. This close-range transmission is the most common way the virus spreads, particularly in crowded or enclosed spaces.
Aerosol transmission
In addition to larger respiratory droplets, smaller aerosol particles can carry the virus over longer distances and remain suspended in the air for extended periods, especially in poorly ventilated indoor environments. Aerosol transmission is thought to be a significant factor in superspreader events and situations where people spend prolonged periods in close proximity, such as in restaurants, gyms, and places of worship.
Fomite transmission (surface transmission)
The virus can also spread through contact with contaminated surfaces or objects, known as fomites. If a person touches a surface that has been contaminated with the virus and then touches their face, especially the eyes, nose, or mouth, they may become infected. While this mode of transmission is considered less common than direct respiratory transmission, it has still contributed to the spread of the virus, particularly in settings like hospitals and households.
Direct contact
Direct physical contact with an infected person, such as shaking hands, hugging, or kissing, can facilitate the transfer of the virus, especially if it leads to the exchange of respiratory droplets or contact with contaminated surfaces. This form of transmission is particularly relevant in household settings and among close contacts.
Vertical transmission (Mother-to-Child)
Although relatively rare, there have been reports of vertical transmission of SARS-CoV-2 from a pregnant woman to her fetus. This can occur during pregnancy, childbirth, or through breastfeeding, though the exact mechanisms and risks are still being studied.
Factors influencing contagiousness
The contagiousness of SARS-CoV-2, often measured by the basic reproduction number (R0), is influenced by several factors:
R0 (Basic Reproduction Number)
The R0 represents the average number of people that one infected individual is likely to transmit the virus to in a fully susceptible population. Early estimates of the R0 for SARS-CoV-2 ranged from 2 to 3, meaning that each infected person could potentially spread the virus to two or three others. This makes SARS-CoV-2 more contagious than seasonal influenza but less contagious than measles, which has an R0 of 12 to 18.
Viral load and shedding
The amount of virus present in an infected person’s respiratory secretions, known as the viral load, plays a significant role in transmission. Higher viral loads, especially in the upper respiratory tract, increase the likelihood of spreading the virus to others. Viral shedding, the process by which the virus is expelled from the body, can begin even before symptoms appear (pre-symptomatic transmission) and continues for several days during the course of the illness. Asymptomatic carriers, who do not show symptoms but still carry the virus, also contribute to the spread, complicating efforts to control transmission.
Variants of concern
The emergence of SARS-CoV-2 variants has affected the virus’s contagiousness. Certain variants, such as the Alpha, Delta, and Omicron variants, have shown increased transmissibility compared to the original strain. Mutations in the spike protein, particularly in the receptor-binding domain (RBD), have enhanced the virus’s ability to bind to the ACE2 receptors on human cells, facilitating easier and more efficient entry into cells. This has led to higher R0 values for some variants and has driven surges in cases even in populations with high vaccination coverage.
Environmental factors
Environmental conditions, including temperature, humidity, and ventilation, influence the transmission of SARS-CoV-2. The virus is more stable in cooler and less humid conditions, which may contribute to higher transmission rates during colder months. Poor ventilation in indoor settings allows viral particles to accumulate in the air, increasing the risk of airborne transmission, particularly during activities like singing, shouting, or exercising.
Superspreader events
Superspreader events, where a single infected individual transmits the virus to a large number of people, have played a significant role in the spread of COVID-19. These events typically occur in crowded settings where many people are in close contact for extended periods, such as at religious gatherings, weddings, conferences, and public transportation. Factors like the timing of the event (e.g., when the index case is most infectious), the environment (e.g., indoor, poorly ventilated spaces), and the nature of interactions (e.g., singing, shouting) can all contribute to these events.
Transmission dynamics
The transmission dynamics of SARS-CoV-2 have been shaped by the interplay of biological, social, and environmental factors:
Asymptomatic and pre-symptomatic transmission
A significant challenge in controlling COVID-19 is the ability of individuals who are asymptomatic (showing no symptoms) or pre-symptomatic (not yet showing symptoms) to transmit the virus. Studies have estimated that a substantial proportion of COVID-19 transmissions occur from people who are not showing symptoms, making it difficult to identify and isolate infectious individuals in time to prevent further spread.
Infectious period
The infectious period for SARS-CoV-2 typically begins 2 to 3 days before the onset of symptoms and continues for about 7 to 10 days after symptoms appear. However, the duration of infectiousness can vary depending on the severity of the illness and the immune status of the individual. Immunocompromised individuals or those with severe illness may shed the virus for longer periods.
Household transmission
Transmission within households has been a major driver of the pandemic. The close and prolonged contact among household members creates an environment where the virus can spread easily. Household secondary attack rates, which measure the likelihood of transmission from an infected individual to others in the same household, have been estimated to be around 10-30%, depending on various factors such as the number of people in the household, their age, and their adherence to preventive measures like mask-wearing and isolation.
Preventive measures and their effectiveness
To curb the transmission of SARS-CoV-2, a variety of preventive measures have been implemented globally:
Physical distancing
Maintaining a physical distance of at least 1 to 2 meters (3 to 6 feet) from others, particularly in public places, reduces the risk of respiratory droplet transmission. Physical distancing has been one of the most effective non-pharmaceutical interventions (NPIs) in reducing the spread of COVID-19, especially in combination with other measures.
Mask-wearing
Masks, particularly those that cover the nose and mouth, are effective in reducing the transmission of respiratory droplets. Widespread mask usage has been associated with lower transmission rates, especially in settings where physical distancing is difficult to maintain. Masks are especially important in indoor settings and in situations where close contact with others is unavoidable.
Hand hygiene
Regular handwashing with soap and water, or using alcohol-based hand sanitizers, is crucial in reducing the risk of fomite transmission. Good hand hygiene practices help prevent the transfer of the virus from contaminated surfaces to the face, where it can enter the body through the mucous membranes.
Ventilation
Improving ventilation in indoor spaces reduces the concentration of viral particles in the air, thereby lowering the risk of airborne transmission. Strategies such as opening windows, using fans, and employing air filtration systems have been recommended to enhance ventilation in homes, workplaces, and public buildings.
Vaccination
The development and distribution of COVID-19 vaccines have been pivotal in controlling the spread of the virus. Vaccines, particularly those targeting the spike protein of SARS-CoV-2, have been shown to reduce the severity of illness, decrease viral load, and lower the risk of transmission. Vaccination not only protects individuals but also contributes to community-level immunity, making it harder for the virus to spread.
Quarantine and isolation
Quarantine (for those who have been exposed to the virus) and isolation (for those who are infected) are critical measures to prevent the spread of the virus to others. These practices help break the chain of transmission by ensuring that potentially infectious individuals do not come into contact with others during their most contagious period.
Vaccines against COVID-19
Vaccines have played a crucial role in the global effort to control the COVID-19 pandemic, reducing the severity of the disease, decreasing hospitalizations and deaths, and helping to curb the spread of the virus. The rapid development and deployment of COVID-19 vaccines represent one of the most significant achievements in public health history.
Overview of vaccine types
There are several types of COVID-19 vaccines, each utilizing different technologies to stimulate an immune response:
mRNA Vaccines: {Pfizer-BioNTech (BNT162b2), Moderna (mRNA-1273)}
These vaccines use messenger RNA (mRNA) to instruct cells in the body to produce a protein that mimics the spike protein of SARS-CoV-2. The immune system recognizes this protein as foreign and produces antibodies against it. If the vaccinated person is later exposed to the actual virus, the immune system is primed to respond quickly, neutralizing the virus. Clinical trials have shown that mRNA vaccines have an efficacy of about 94-95% in preventing symptomatic COVID-19. They have also been effective in reducing severe disease and hospitalization.
Viral vector vaccines: {Oxford-AstraZeneca (Vaxzevria), Johnson & Johnson (Ad26.COV2.S), Sputnik V}
These vaccines use a harmless adenovirus (a common cold virus) as a vector to deliver the genetic material coding for the spike protein of SARS-CoV-2 into cells. The cells then produce the spike protein, triggering an immune response. Viral vector vaccines have shown efficacy ranging from 60-80% in preventing symptomatic COVID-19, with high efficacy in preventing severe disease and hospitalization.
Protein subunit vaccines: {Novavax (NVX-CoV2373)}
These vaccines contain harmless pieces of the spike protein (protein subunits) rather than the whole virus. When injected, the immune system recognizes these proteins as foreign and generates an immune response. Protein subunit vaccines have shown efficacy rates of around 90% in clinical trials, particularly in preventing symptomatic COVID-19.
Inactivated or live attenuated vaccines: {Sinopharm (BBIBP-CorV), Sinovac (CoronaVac), Covaxin}
Inactivated vaccines use a version of the virus that has been killed or inactivated so that it cannot cause disease. Live attenuated vaccines use a weakened form of the virus. Both types stimulate an immune response without causing illness. Inactivated vaccines have shown varying levels of efficacy, generally ranging from 50-80%, depending on the vaccine and the population studied.
Vaccine development and approval
The development of COVID-19 vaccines was unprecedented in speed, thanks to global collaboration, advances in vaccine technology, and significant financial investments. The typical vaccine development timeline, which can take 10-15 years, was compressed into less than a year for COVID-19 vaccines. Vaccine candidates underwent rigorous testing in preclinical studies and three phases of clinical trials. These trials involved tens of thousands of participants and were designed to assess the safety, efficacy, and immunogenicity of the vaccines.
Emergency Use Authorization (EUA): Given the urgent need to control the pandemic, regulatory agencies such as the U.S. Food and Drug Administration (FDA), European Medicines Agency (EMA), and others around the world granted Emergency Use Authorization (EUA) for several COVID-19 vaccines. EUA allows vaccines to be distributed and administered during public health emergencies, based on available data showing that the benefits outweigh the risks.
Full approval: As more data became available from ongoing clinical trials and real-world studies, some vaccines, like Pfizer-BioNTech and Moderna, received full approval from regulatory agencies, signifying their long-term safety and efficacy.
Efficacy and effectiveness
Vaccine efficacy refers to the percentage reduction of disease in a vaccinated group compared to an unvaccinated group under ideal conditions (e.g., in clinical trials). The initial trials of COVID-19 vaccines showed high efficacy rates, especially against severe disease, hospitalization, and death. Effectiveness refers to how well a vaccine performs in real-world conditions. Studies have shown that COVID-19 vaccines are highly effective in reducing the incidence of severe disease and death, even against various SARS-CoV-2 variants. However, the effectiveness can vary based on factors such as the specific variant, the time since vaccination, and the population being studied.
Booster doses: As the pandemic progressed, it became evident that immunity from the initial vaccine doses waned over time, particularly against emerging variants like Delta and Omicron. Booster doses were recommended to enhance and prolong immunity, with studies showing that boosters significantly improve protection against both infection and severe outcomes.
Vaccine distribution and global access
Global vaccination campaigns: The rollout of COVID-19 vaccines has been one of the largest vaccination campaigns in history. High-income countries were able to secure vaccine doses early, leading to rapid vaccination rates. However, low- and middle-income countries faced significant challenges in accessing vaccines, leading to global disparities in vaccination coverage.
COVAX initiative: The COVAX initiative, co-led by the World Health Organization (WHO), Gavi, the Vaccine Alliance, and the Coalition for Epidemic Preparedness Innovations (CEPI), was established to ensure equitable access to COVID-19 vaccines. COVAX aimed to distribute vaccines to countries that could not afford them, focusing on vaccinating high-risk populations such as healthcare workers and the elderly.
Cold chain requirements: Some COVID-19 vaccines, particularly mRNA vaccines like Pfizer-BioNTech, require ultra-cold storage temperatures, posing logistical challenges for distribution, especially in regions with limited infrastructure. Other vaccines, like AstraZeneca and Johnson & Johnson, have less stringent storage requirements, making them more accessible in low-resource settings.
Vaccine hesitancy and public perception
Vaccine Hesitancy: Despite the proven safety and efficacy of COVID-19 vaccines, vaccine hesitancy has been a significant barrier to achieving high vaccination coverage. Vaccine hesitancy is influenced by factors such as misinformation, distrust in governments and pharmaceutical companies, concerns about vaccine safety, and cultural or religious beliefs. Public health campaigns, community engagement, and transparent communication have been critical in addressing vaccine hesitancy. Efforts to provide accurate information, debunk myths, and build trust in vaccines have been ongoing, with varying levels of success in different regions.
Vaccine safety and side effects
Common side effects:
Most COVID-19 vaccines are associated with mild to moderate side effects, such as pain at the injection site, fatigue, headache, muscle pain, chills, fever, and nausea. These side effects typically resolve within a few days and are a sign that the immune system is responding to the vaccine.
Rare side effects:
In rare cases, more serious side effects have been reported, including allergic reactions (anaphylaxis) and myocarditis (inflammation of the heart muscle) following mRNA vaccination. The Johnson & Johnson vaccine was associated with a rare but serious condition called thrombosis with thrombocytopenia syndrome (TTS), which involves blood clotting with low platelet counts. Despite these rare events, the benefits of vaccination far outweigh the risks.
Vaccine safety is continuously monitored through various surveillance systems, such as the Vaccine Adverse Event Reporting System (VAERS) in the U.S., and similar systems in other countries. These systems help detect rare side effects and ensure that the benefits of vaccines continue to outweigh the risks.
Herd immunity against COVID-19
Herd immunity is a form of indirect protection from infectious diseases that occurs when a significant portion of a population becomes immune to the disease, either through vaccination or previous infection. When a large percentage of the community is immune, the spread of the disease is slowed or even stopped because there are fewer susceptible individuals for the pathogen to infect. The herd immunity threshold (HIT) is the proportion of the population that needs to be immune to a disease for herd immunity to be achieved. The HIT is influenced by the basic reproduction number (R0), which represents the average number of people one infected person will transmit the virus to in a fully susceptible population. The higher the R0, the greater the proportion of the population that must be immune to achieve herd immunity. The formula to estimate the HIT is,
𝐻𝐼𝑇 = 1 – 1/𝑅0
For example, if the R0 of SARS-CoV-2 is estimated to be around 3, the HIT would be approximately 67%, meaning that about two-thirds of the population would need to be immune to achieve herd immunity.
Vaccination is the most effective and safest way to achieve herd immunity. By immunizing a large portion of the population, the spread of the virus is reduced, protecting those who cannot be vaccinated, such as individuals with certain medical conditions, or those who do not respond well to vaccines.
Challenges in achieving herd immunity against COVID-19
Variants of Concern: The emergence of SARS-CoV-2 variants with increased transmissibility, such as Delta and Omicron, has raised the R0, thus increasing the HIT required for herd immunity. For instance, if the R0 of a variant is 5, the HIT would be 80%. Variants with mutations that allow them to partially evade immunity, either from vaccines or previous infection, further complicate the achievement of herd immunity.
It should be remembered here that, immunity to SARS-CoV-2, whether from natural infection or vaccination, can wane over time. This reduction in immunity means that people who were previously protected might become susceptible again, making it difficult to maintain herd immunity over the long term. Booster doses have been introduced to help maintain immunity, but this requires ongoing public health efforts. Vaccine hesitancy has been a significant barrier to achieving high vaccination coverage in many regions. Misinformation, distrust in authorities, concerns about vaccine safety, and cultural factors have all contributed to lower-than-necessary vaccination rates, preventing populations from reaching the HIT.
There are significant disparities in vaccine distribution and access between high-income and low- and middle-income countries. Many lower-income countries have struggled to vaccinate even their most vulnerable populations, let alone achieve the levels of coverage needed for herd immunity. This global inequality poses a risk not only to the countries with lower vaccination rates but also to the global community, as ongoing transmission increases the likelihood of new variants emerging. Even with high vaccination coverage, human behavior plays a critical role in the spread of the virus. Factors such as adherence to public health measures (e.g., mask-wearing, social distancing), the relaxation of restrictions, and the return to pre-pandemic activities can influence the ability to achieve and maintain herd immunity.
Herd immunity and COVID-19 strategies
Vaccination campaigns
Achieving herd immunity through vaccination has been the cornerstone of most countries’ strategies to combat COVID-19. Mass vaccination campaigns aim to immunize as many people as possible, particularly focusing on vulnerable populations such as the elderly, those with underlying health conditions, and healthcare workers.
Booster programs
To address waning immunity and the challenge posed by variants, many countries have implemented booster vaccination programs. These boosters are designed to enhance and extend the duration of immunity, potentially helping to achieve and maintain herd immunity.
Alternative approaches
Some strategies have focused on living with the virus rather than eradicating it, acknowledging that achieving herd immunity may not be feasible in the short term. This approach emphasizes protecting vulnerable populations, managing healthcare capacity, and minimizing severe disease and death rather than eliminating transmission entirely.
The Future of Herd Immunity in the Context of COVID-19
Endemicity: As it becomes clear that achieving herd immunity against COVID-19 may be challenging due to the factors mentioned above, there is growing recognition that SARS-CoV-2 might become endemic. An endemic virus is one that continues to circulate in the population but at lower, more manageable levels. In this scenario, periodic outbreaks may occur, but the widespread immunity (through vaccination and natural infection) could help prevent large-scale epidemics and severe outcomes. Even if herd immunity in the strictest sense is not achievable, ongoing vaccination efforts are crucial. Vaccination can help reduce the severity of illness, prevent healthcare systems from being overwhelmed, and protect the most vulnerable members of society. Achieving a high level of immunity worldwide is essential to controlling the pandemic. This requires global cooperation in vaccine distribution, manufacturing, and sharing of knowledge and resources. The COVAX initiative and other global efforts aim to ensure that all countries have access to vaccines, which is vital for managing the pandemic on a global scale.
Conclusion
The COVID-19 pandemic has catalyzed an unprecedented amount of scientific research, leading to rapid advancements in our understanding of the virus, its transmission, and strategies for prevention and treatment. However, many questions remain unanswered, and ongoing research is essential to fully understand and control the disease. Future research on COVID-19 will span a wide range of fields, from basic virology and immunology to public health, social sciences, and environmental studies. By addressing the many unanswered questions and challenges posed by the pandemic, this research will not only help us better understand COVID-19 but also prepare us for future global health threats.
References
References are available in the hardcopy of the website “Periobasics: A Textbook of Periodontics and Implantology”.
Periobasics: A Textbook of Periodontics and Implantology
The book is usually delivered within one week anywhere in India and within three weeks anywhere throughout the world.
India Users:
International Users: